A Quantitative Analysis of the Lead Leg Block and its Contributions to Velocity
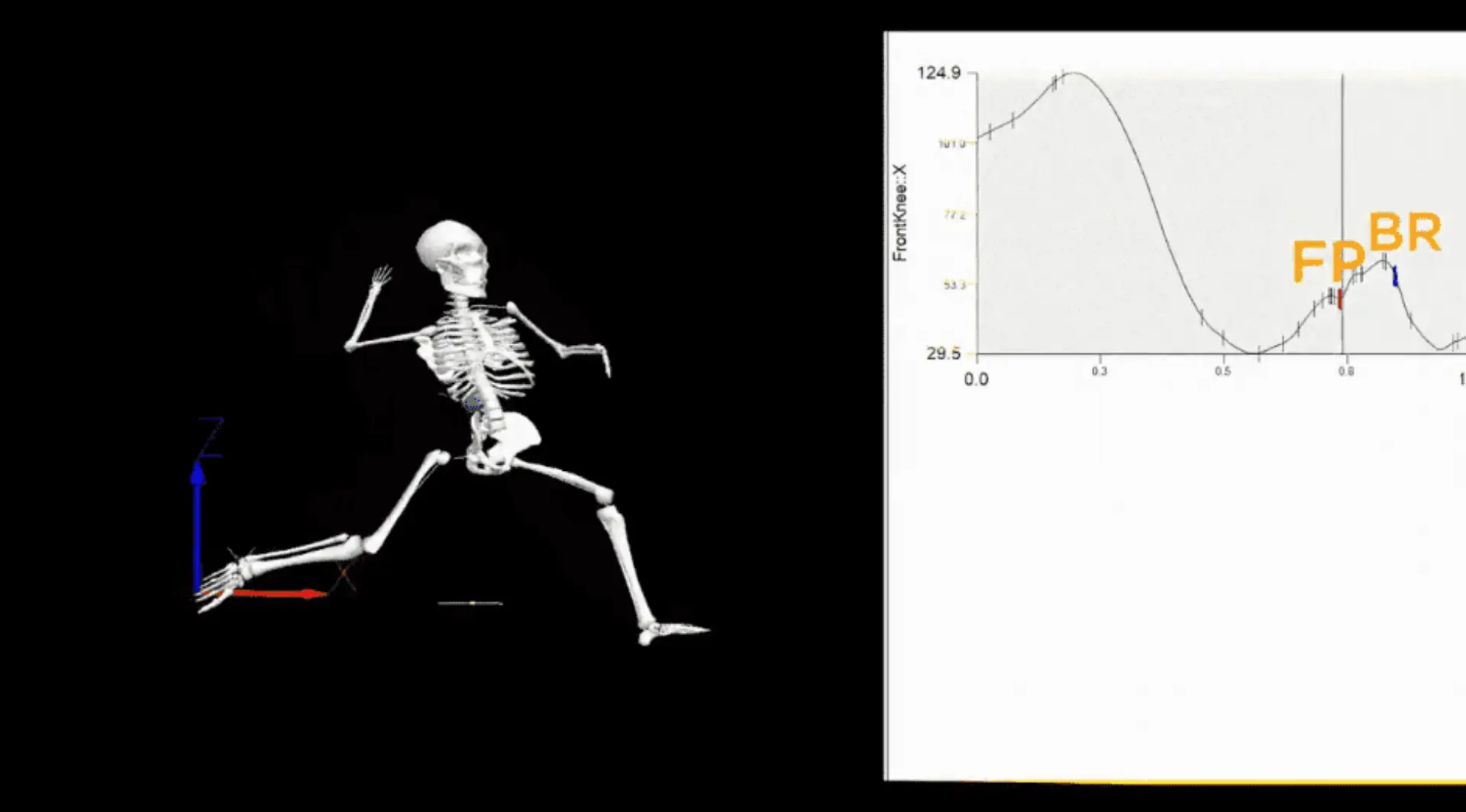
Summary
Throughout this post we’ll take a closer look at various aspects of the lead leg block in pitching, looking through our biomechanics database to better describe how the lead leg block works to enable pitchers to throw harder. To briefly summarize the main findings:
-Athletes with more knee extension after foot plant throw harder (even within their own throws) on average.
-Athletes with more COG deceleration after foot plant throw harder on average
-COG deceleration correlates with front knee extension somewhat
-The block driving faster or more total pelvis rotation after footplant does not distinguish harder and softer throwers
-Both max vertical and anterior/posterior Ground Reaction Forces correlate with velocity – with the direction of that max force varying by athlete and not being a significant driver on average of pitch velocity
– Most of the relevant lead leg positions at footplant do not correlate notably with pitch velocity or the quality of the block.
Intro
With our last blog on the lead leg block being from 2015, we’ve since integrated our biomechanics lab into our training process for all athletes training in-gym and collected thousands of throws. We have a unique collection of high fidelity data with a plethora of pitchers from various skill levels and velocities up to 100mph in the dataset to analyze what the hardest throwers do well – which then informs our training processes.
We have touched on blocking briefly in other blogs since then, such as our initial SPM blog, however we’re dedicating space here to digging in with more detail- as well as examining the database with the throwers who’ve assessed in the lab since then. We are looking at various discrete metrics here, so this won’t be as sophisticated technically as the full signal analysis in the SPM blog, but is sufficient to address a variety of specific questions and hypotheses of interest.
We investigate three major kinematic components of blocking: knee extension, linear center of gravity velocity deceleration, and pelvis rotation. Then we’ll review our force plate data to consider the ground reaction forces. To wrap up, we consider how a pitcher’s landing position at foot-plant factors into how well an athlete is able to block across the kinematic components considered.
Knee Extension
How important is extending the front knee?
To define knee extension, we look at the angle of the front knee at foot-plant and at ball release and calculate the difference between them. A positive value indicates that the knee extends from FP to BR.
Comparing the throws from each athlete with a repeated measures correlation, we get a moderately strong (in the context of biomechanics correlations to velocity being fairly low across the board) correlation of r = 0.29. This is pretty similar to the r = 0.27 comparing front knee extension and velocity between different throwers. That is, both athletes who extend their knee more throw harder than athletes who extend their knees less and athletes throw harder when they extend their knee more on specific throws of theirs – on average.
A couple examples to illustrate what this looks like:
Gains 34° of knee extension.
Loses 6° of extension.
A nonlinear model fits the data better, with the key insight being the steep drop off at the low end – which is to say that athletes who sink into a lot more flexion at BR relative to where they were at FP have the most to gain by improving. At the high end, it does trend up again slightly, which seems to be driven by a couple athletes who throw moderately hard with the most knee extension.
If extending the knee is good, does that mean flexing the knee at the start is especially bad?
For this, we looked at the most flexed position an athlete reaches between foot plant and ball release and looked at how much more flexed this was relative to the knee angle at foot-plant – to consider how bad it is to sink into the lead leg more after foot-plant.
This is strongly related to how much the athlete’s able to extend their knee between FP and BR- which should come as no surprise (with extension being the inverse of flexion and us considering a portion of the same time period).
Put another way, if you leak into more flexion after foot-plant, it’s tougher to have your knee get more extended than it was at footplant. This makes sense, since we’re measuring extension against the value at foot-plant, so if you sink into 5 degrees of additional flexion in the time right after foot-plant, you now have less time to extend the knee before ball release and you have an additional 5 degrees you need to extend through just to get back to the starting point – much less get more extended.
A couple examples illustrating how sinking into more flexion can differ from the extension from FP to BR to illustrate what each specifically looks like on its own, though they are generally related to each other.
Visual for what not gaining flexion looks like (-0.78°) – with minimal extension from FP to BR (7°)
Visual for what gaining flexion looks like (-12°) – with extension from FP to BR (16°)
Looking at the relationship between sinking into additional flexion with velocity, we see a weaker trend than with using knee extension from FP to BR – with most of the observed trend being that it’s bad to sink into a lot of flexion (plot below). This relationship is notably weaker than for extension especially intra-subject where the r = 0.13 as opposed to 0.29. That is, looking at an athlete’s throws individually, the ones where they extend their knees more tend to correspond to the ones they threw harder more strongly than the ones where they sink into more flexion do – though there is a weak relationship for sinking into flexion as well.
Given the relationship between extending the knee from FP to BR and sinking into additional flexion after FP, it makes sense to ask if adding in how much flexion an athlete sinks into affects their velo after you account for the effect it has on their total amount of extension that they gain. If we throw them in a combined multiple regression model, we see only a marginal gain in model fit relative to the model with just the difference in knee extension angle (r^2 = 10.34 vs 10.21), suggesting that the relationship above between avoiding sinking into flexion and throwing harder is related to how avoiding sinking into more flexion makes it easier to extend the knee more – or some shared mechanism that affects both – rather than some additional value it may provide on its own.
To apply that interpretation, if you sink into lots of flexion, it’s going to make it incredibly difficult to extend your knee at all. However, if you sink into a little bit of flexion – but are able to extend your knee (like the second example from above), that’s likely less cause for concern and likely preferable to holding the same angle the entire time from foot-plant to ball-release.
Additionally, heavier pitchers (r = -0.15) and pitchers who move faster (r = -0.21) both on average sink into very slightly more flexion which is also interesting to observe.
Well what about how fast you extend your knee?
To touch on front knee extension angular velos as well, we start with the three most commonly calculated points: at foot-plant, at ball release, and the max value.
Max Front Knee Extension Angular Velocity and Front Knee Extension Angular Velocity at BR are strongly correlated with each other – so are likely redundant.
Both max and at BR correlate decently with velocity – and are similar in strength in regards to correlation with velocity (r = 0.25 inter-subject, r = 0.20 intra-subject).
Thus, these likely provide some information and are useful for assessing blocks, and either one could be used interchangeably. Athletes who have larger differences between the two – who peak their knee extension velo before BR – don’t on average throw notably harder or slower (r = -0.065 with velocity).
Looking at front knee extension angular velo at foot-plant (how quickly they’re extending their knee as they plant their foot) – we see a much weaker relationship (r = 0.05 intra- and inter- subject). A quadratic fit is a smidge better (where extending or flexing the knee rapidly going into foot-plant are both not optimal) – but even so, still fairly weak to the point that I wouldn’t put much weight on it.
Note that this is weakly correlated with some of the other front knee metrics discussed above: r = ~0.2 with front knee extension from FP to BR (inter- and intra- athlete), r – ~0.15 with max front knee extension angular velo (inter- and intra-athlete), r = 0.13 inter- and r=-0.01 intra with front knee extension angular velo at br. Which is to say that it does relate some to those other measures – which may be part of why it has a small amount of predictive power.
A couple examples to illustrate how front knee extension angular velo at FP can differ from front knee extension from foot-plant to ball-release to show what it’s specifically measuring:
Gains 35° of extension but from -238°/sec ext velo at FP
Gains 35° of extension but from -238°/sec ext velo at FP
Killing Linear Momentum
How important is decelerating center of gravity movement towards the plate?
Another main component of the block is decreasing linear momentum and transferring that energy up the chain. Following on Kyle Lindley’s idea for a change in momentum metric , we calculated a metric for the change in COG velocity in the direction between the rubber and the plate, from its max to the minimum value between FP and BR.
Note that we see a trend where athletes who move down the mound faster are able to decelerate more – since they have a greater peak value to work off of. We can control for this relationship and get how much a particular athlete slows down their COG relative to how much we’d expect given their peak COG. This is pretty similar to the percentage metric Lindley first calculated – though we take residuals rather than using a ratio to avoid the assumptions ratios require, and don’t multiply by weight to avoid introducing any explanatory power since heavier athletes will on average throw harder.
You could hypothesize that stopping COG immediately after foot-plant is the most important and looking all the way up till ball-release is a relatively long time period to look at. If we look at only the first half of the window from FP to BR (so if, as an example, FP is at frame 220 and BR is at 240, looking at frames 220-230) to get an idea of how quickly athletes slow their COG shortly after foot-plant, this metric is less predictive of velocity (r = 0.15 inter-athlete and r = 0.04 intra-athlete).
That metric for COG difference during the whole period from FP to BR does relate some with front knee extension – though notably not perfectly. As you can see in the plots below, for a specific front knee extension value there’s a spread in terms of the COG difference metric:
A couple examples showing how these don’t always line-up (that I thought were interesting):
Knee extension of 6, but has the top value in terms of killing max COG velo- residual of 0.6.
Front knee extension of 36, but COG decel residual of -0.32 which is towards the lower end.
Note that ideally we’d separate out the deceleration of the COG of the lower half from the upper half. With the COG being the average weight position of all segments, this metric doesn’t account for athletes who slow down their lower half and use that to propel their upper half towards the target more than others (as the examples above illustrate).
Pelvis Rotation and Rotational Acceleration
How important is finishing Pelvis Rotation and increasing Pelvis Rotational Velo after Foot-plant?
The third major component of the block that is often talked about (along with converting the linear momentum up the chain, and the knee extending) is driving/finishing rotation of the pelvis:
To investigate this, we look at how much the pelvis rotates between foot plant and ball release (so pelvis angle at BR – pelvis angle at FP) – which does not notably relate to pitch velo (r = -0.07 inter- and r = 0.10 intra-athlete).
Note that this is strongly related with pelvis rotation at foot-plant (plot below). That is athletes who land with a more closed pelvis on average rotate their pelvis more between foot-plant and ball release. Controlling for this relationship (to look at athletes who rotate their pelvis more than we’d expect given their pelvis rotation at footplant) doesn’t strengthen the relationship with velocity (r = -0.06 inter- and r= 0.10 intra-athlete again).
Looking at pelvis rotational velo rather than just the positions, we compared pelvis rotational velo at FP with the max after FP (technically 1 frame after to avoid any overlap for people who only decelerate after) up to BR.
We see a small portion (7.8%) of athletes who’s max pelvis rotational velo after FP is no greater than their pelvis rotational velo at FP, with guys on average increasing their pelvis rotational velo by 136°/sec from FP to their max before BR.
This does not correlate notably with velo (r = -0.07 inter-subject and r = -0.01 intra-subject)
This does relate moderately with pelvis rotation at FP – where guys who have more closed pelvises at foot plant gain more pelvis rotational velo by ball release (some of which may be coming from having slower rotating pelvises at FP and some from having a larger arc to accelerate through).
To provide a couple visual examples of what outlier pelvis rotational velo gains look like – for reference:
Pelvis Rot Velo Gain of 425 – 98th percentile
Pelvis Rot Velo Gain of -36 – 1st percentile
We can similarly look at front hip internal rotation positional changes and front hip internal rotation velo since that captures rotation around the front hip that may provide more context in regards to femur positioning than just looking at global pelvis rotation, but we get similarly weak and non-notable results for change in front hip IR from BR to FP (r = 0.007 inter- and r = 0.09 intra-athlete) and max hip ir velo from between FP and BR (r = -0.03 inter- and r= 0.01 intra-athlete).
Evaluating Blocks
How should we weigh the different components when evaluating blocks given that there’s some overlap between them?
All that being said – how should we account for those different components when evaluating a block? To answer this question we built a multiple regression model predicting velocity taking in the variables from the different components of blocking. This allows us to calculate the best linear formula for predicting velocity using different variables. Thus, it provides a way to calculate how we’d expect different variables to contribute to velocity while controlling for the other input variables, and therefore identify what unique information each variable offers.
With Front Knee Extension from FP to BR and the COG decel metric correlating the most with velocity on their own, it’s not surprising that they provided the largest increase in model explanatory power. Adding front knee extension angular velo at BR and the max value on top of these two didn’t increase performance – so it seems that the information those would add is already captured in those two initial metrics. Similarly, we also tested out adding pelvis rotation related metrics to see if they would show up as more notable when controlling for the effect of the other components of blocking, and it still came up as not adding much.
Looking at the top blocks by this combined metric (hereby dubbed the composite block metric), we see the top performers are those with the most lead knee extension with some guys getting a bit more credit because they slow down their COG more effectively, though they also straighten their knees decently as well.
Top composite block score
2nd highest
8th best, which this pitcher achieves by having elite COG decel as well as good lead knee extension
Ground Reaction Forces
How do ground reaction forces relate to those kinematic measures of the block and to velocity?
With the addition of force plates to our lab and processing pipeline, we have the ability to dissect the lead leg block even further beyond what you can pick up from video and motion capture – by looking at ground reaction forces.
While we’ve done a couple case studies before looking at force plate data such as in these blogs from 2016 and 2017; none of these have anywhere close to the sample of force plate data we’ve been able to collect with the set-up in our lab now – where we have more than 800 unique sessions with force plate data.
Looking at lead leg ground reaction force broken up into X/Y/Z components for the anterior-posterior/lateral/and vertical components, we see that the anterior-posterior and vertical components correlate to velocity with similar strength (r = 0.38 for x, and r = 0.44 for z) – whereas the y component (going between first and third) does not as strongly (r = 0.19).
However all of these are inflated by the fact that heavier athletes throw harder across the sample of HS/College/Pro athletes we have here – so that confounds the relationship observed here.
After we control for body-weight, the correlation for all three of the components drops off some, but the vertical and anterior-posterior components winds up in a similar neighborhood to what we observed for the key kinematic measures of the lead leg block (r = 0.19 for x, r = 0.23 for z, r = 0.1 for y – and r = 0.25 for the max magnitude considering all three components).
An interesting result here is that the X and Z components both correlate with similar strength to velocity – whereas some of the previous literature had found the X component to matter more – in smaller samples. If we consider the direction of the force (where 90 degrees is all vertical force, and 0 degrees is all horizontal force with no vertical component), we see no correlation to velo. That is, on average there does not seem to be a general recommendation for what angle you should aim to put force into the ground across all athletes, but merely that putting more force into the ground with your lead leg is better.
Comparing the max Lead Leg force into the ground with the Block Composite Score calculated from the different kinematic areas we see that they are correlated, but that correlation isn’t all that high in context of what you might expect (r = 0.26). Which is to say that the kinematic measures give us some idea of the force put into the ground, but there’s more detail that we’re able to get at leveraging the force plates that doesn’t show up in the kinematic measures – giving us another measure tool for assessing and diagnosing athlete’s training needs.
Positioning
How does landing positioning affect this composite block metric and the different blocking metrics?
The table above shows the inter-subject (comparing different athletes) and intra-subject (comparing throws from each athlete) correlations comparing different metrics of interest for blocking with foot-plant positioning metrics. Relationships I think may be of interest highlighted in orange. The relationships between these various landing position metrics and our composite block metric are all very weak – which suggests to me that landing position is not the primary determinant of an athlete’s blocking ability.
We considered 4 positional metrics at foot-plant – front knee flexion, front ankle dorsi/plantar flexion, pelvis rotation, and hip internal/external rotation. For each of these, I took a look at how they compared to ball velo, the composite block metric, detailed above, and then front knee extension from FP to BR, front knee flexion from FP to the min after FP, COG decel after FP, and Max Front Knee Extension Angular Velo which were the main metrics discussed above.
Front Knee Flexion at FP
First we consider the angle of the knee at foot-plant, since it’d seem that how this angle is initially set would significantly affect how the block works. Quick visual demonstration of the variability we see:
35° on left (3rd percentile) 68° on right (98th percentile)
Thus a larger number is a more flexed knee at foot-plant. Most of the relationships come back as non-notable when combining the inter- and intra- athlete analysis.
The one relationship that is notable in both is the relationship between front knee flexion and sinking into additional flexion after foot-plant – we get r = 0.26 comparing different session-level averages per athlete and r = 0.2 comparing all throws within each athlete.
This relationship indicates that having a more bent knee at foot-plant leads to sinking into flexion less after foot-plant. That might seem counterintuitive at first, but as the knee is already more bent, it’s less likely to bend even more. Furthermore, it’s possible that the athlete has a particular joint angle that they’re stronger in – so by landing in a more flexed position – that could theoretically be more advantageous for extending out of.
Front Ankle Dorsi-flexion at FP
Front ankle dorsi/plantar flexion is going to be decently related to front knee flexion angle (r = 0.40), but I thought it would be interesting to consider as well, since they don’t line up 100%.
Ankle dorsiflexion at 59° Ankle dorsiflexion at 91°
Here, most of the relationships come back non-notable, so it doesn’t seem to be a huge determiner of someone’s ability to block, especially since there’s no notable correlation to ball velo or the composite block metric.
Pelvis Rotation at FP
After looking at the lead leg positions specifically, we consider pelvis rotation (axially, so omitting anterior/posterior tilt and lateral tilt of the pelvis in this analysis) next – as if the pelvis is open enough is often considered a factor in blocking ability.
A quick visual at high and low pelvis rotation numbers to illustrate:
Pelvis Angle at 15° Pelvis Angle at 65°
There’s a negligible relationship between pelvis rotation at FP and ball velo (r = 0.08 between-athlete, r = 0.02 within-athlete) and pelvis rotation at FP and the composite block metric (r = -0.04 inter-athlete, and r = 0.03 intra-athlete) – which immediately qualifies the importance of having the pelvis open at foot-plant for blocking. If getting the pelvis open was a prerequisite for being able to block, you’d expect harder throwers and better blockers to get their pelvis more open – which on average they do not.
We do see that athletes who land with more open pelvises on average sink less into flexion after footplant (as well as intra-subject – where athletes sink less into flexion after foot-plant on throws where their pelvis is more open). The correlations here are the strongest of any of these landing position correlations (r = 0.34 inter-, and r= 0.45 intra-subject). By landing with the pelvis more open, an athlete may avoid sinking into more flexion but in the process also reduce the amount of time they have for their leg to impart force into the ground before ball release – and thus just be trading off sinking into a little bit of flexion at the expense of having time to put force into the ground.
If someone is sinking into a lot of flexion and not getting any extension, it’s possible that opening the pelvis more up may help, by affording them less time to do so – but I don’t think that fully addresses the issue of lack of extension given the lack of a relationship between pelvis angle and throwing harder or blocking better (as measured by the composite block score)
Hip ER at FP
Last one on the docket is Hip IR/ER. It is a bit more subtle in terms of being able to see it than the other landing position metrics. We have a couple athletes with more extreme values to illustrate:
Low Hip IR/ER at FP of -25° High Hip IR/ER at FP of 46
°
This metric is looking at the rotation of the front femur relative to the pelvis. When looking for it, you can see the athlete on the left have his femur turned inwards in relation to the pelvis as opposed to the athlete on the right who has his turned out.
We see more Hip ER at FP related to sinking into more flexion after FP, and getting less extension of the knee between FP and BR. From looking at athletes with extreme hip ER values (screenshots below) – we can see how being more externally rotated may put an athlete in a disadvantageous position to be able to extend the knee. Nonetheless, as with the other landing position metrics, Hip ER at FP doesn’t relate notably to throwing harder or blocking better in general, so this may be a possible option to explore with athletes with extreme ER, but isn’t something that necessarily weighs heavily when evaluating and troubleshooting an athlete’s blocking ability.
Conclusion
The ability to analyze mechanics from all the pitchers who have come through the lab allows us to rigorously test out a variety of hypotheses to determine what distinguishes hard throwers from slower throwers. We see our hard throwers extending their knees more and faster, putting more force into the ground vertically and horizontally, and slowing their center of gravity down more than the slower throwers on average.
Thus, checking out knee extension provides a valuable check-point when considering an athlete’s mechanics – which relates to the athletes ability to slow down their body and transfer that energy up the chain and put force into the ground. We don’t see the quality of the lead leg block being dictated by the position an athlete lands in – suggesting that the lead leg block is a skill/movement that likely needs to be trained (with drills like rockers, roll-ins and drop-steps) – and won’t necessarily address itself completely just by changing how an athlete moves into foot-plant.
While we’ve aimed to be thorough here, there’s always more areas to investigate. We touched on force plate components in this analysis, but there’s further analysis on the timing of ground reaction forces and the relationship between putting force into the ground and how that coincides with different movements. Besides that, the role of strength and mobility on blocking ability is often talked about and would be interesting to discuss here, but that’s an analysis for another time.
Comment section